
The Raman laboratory research program aims to develop technologies at the intersection of biochemistry, microbiology, computation, and engineering to understand the fundamental principles of biomolecular and cellular systems. Our laboratory takes a systems and synthetic biology approach to understanding and engineering biological systems at protein-wide and genome-wide scales. A common theme that underpins all projects in our laboratory is devising experimental screens to make thousands to millions of mutational perturbations and measurements in biological systems in a single-pot experiment. Such highly multiplexed (pooled) screens have three fundamental advantages because they (1) reveal the entire functional landscape of the system, (2) uncover non-intuitive sequence-function relationships, and (3) train machine-learning models to design and engineer new functions.
UNDERSTANDING AND ENGINEERING GENE/GENOME SEQUENCE FUNCTION LANDSCAPES
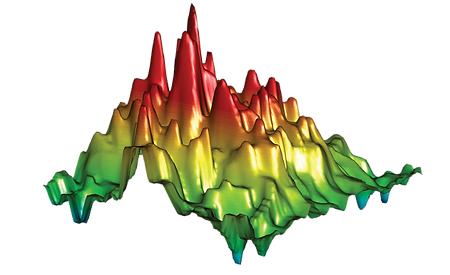
Another common theme in the laboratory is the close alignment of basic science research with translational impact in the real world. We use computational protein design, deep mutational scanning, chip-based DNA synthesis, deep sequencing, and single-cell RNA sequencing as tools to address the following questions.
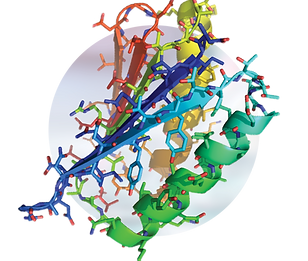
PROTEIN ALLOSTERY
Our goal is to understand the molecular and evolutionary principles of protein allostery and to design novel allosteric biosensors for biotechnological applications. MOLECULAR RULES OF ALLOSTERY: Allostery is regulation from a distance – perturbation at one site of a protein causes an effect at a distant site. Allostery has a central place in biology due to the myriad roles of allosteric proteins in cellular function. We develop a data-driven framework to understand, quantify and predict molecular drivers of protein allostery by combining deep mutational scanning and machine learning. We investigate several bacterial and human allosteric transcription factors as model systems. ENGINEERING ALLOSTERIC BIOSENSORS: Customized protein biosensors to detect a specific small molecule or groups of molecules are a valuable tool for synthetic biology. Allosteric transcription factors (aTF) are widely used as biosensors. However, a major obstacle is a reliance on natural aTFs for biosensing, of which only a small number are well characterized, leaving large chemical classes with no biosensors. We combine computational protein design (Rosetta) with experimental pooled barcoding techniques to evaluate thousands of aTF designs simultaneously to design novel biosensors.

BACTERIOPHAGE HOST INTERACTIONS
Our goal is to investigate how genetic perturbations in phages and hosts influence their mutual interactions and to engineer programmable synthetic phages for biotechnological applications. UNDERSTANDING PHAGE-HOST INTERACTIONS: To elucidate sequence-function relationships in phages, we use deep mutational and metagenomic scanning through phage genome engineering and selection. This allows us to create site-specific mutational profiles of individual phage genes and discover the functions encoded in the viral metagenome by the pooled screening of hundreds of thousands of sequence variants. To understand host factors governing phage activity, we carry out genome-wide host screens to uncover gene and gene networks that regulate phage infectivity. PHAGE-BASED ANTIBACTERIAL THERAPIES: Bacteriophage therapy could be a promising solution to the antibiotic resistance crisis, as evidenced by many recent success stories. However, the use of natural phages has fundamental limitations in efficacy, reliability, scalability and speed. We are developing a new framework by high-throughput precision genome engineering of natural phages (as chassis) to create potent phage variants suitable for therapeutic applications. We are engineering phages against several multi-drug-resistant, urinary tract and food-borne pathogens. We use machine learning models to design novel phage variants with desired properties. PRECISION MICROBIOME EDITING WITH BACTERIOPHAGES: Dysbiosis of the gut microbiome has been linked to many human diseases. An emerging paradigm for the treatment or prevention of gut-linked human disorders is to precisely shift the gut microbiome to a desired, health-promoting state. We are developing phages as tools to enable targeted and precise engineering of the gut microbiome in situ. These phages can either remove or add function to the microbiome.

HUMAN DISEASE VARIANTS
Our goal is to understand the functional impact of variants in disease-relevant human genes using deep phenotyping measurements. Functional genomic studies to characterize cellular and clinical consequences of genetic variants lags far behind the pace of genome sequencing. Only a very small fraction of the millions of currently cataloged missense mutations in the human genome has functional annotation. Using deep phenotyping assays, we aim to measure the cellular impacts of mutations in these genes and to cluster mutants based on transcriptional dysregulation. These studies are intended to enable patient-specific drug targeting

NEW EMERGING THEMES
TRANSPORTERS: Molecular and energetic drivers of transporters, engineering new transporters with customized specificities. GENE DELIVERY SYSTEMS: Design gene delivery particles with tissue targetability and high payload capacity. VACCINE DESIGN: Design of novel vaccine scaffolds with customized epitope presentation